MLCC Flux Entrapment
MLCC short circuit failures on PCBAs are often caused by bending fractures, where internal electromigration shorts develop between opposite electrodes along the fracture. Short circuit failures are also caused sometimes by capacitor manufacturing defects such as knit line failure, firing cracks, or dielectric porosity. But we see more frequently in recent years cases where the capacitor short is external, growing due to electrochemical migration through flux entrapped under the MLCC from terminal to terminal.
Fortunately, our primary method of examination of MLCCs is a microsection of the capacitor as mounted on the PCBA (Fig. A). This orientation is ideal for evaluation of bending fractures. It also affords us a look under the capacitor.
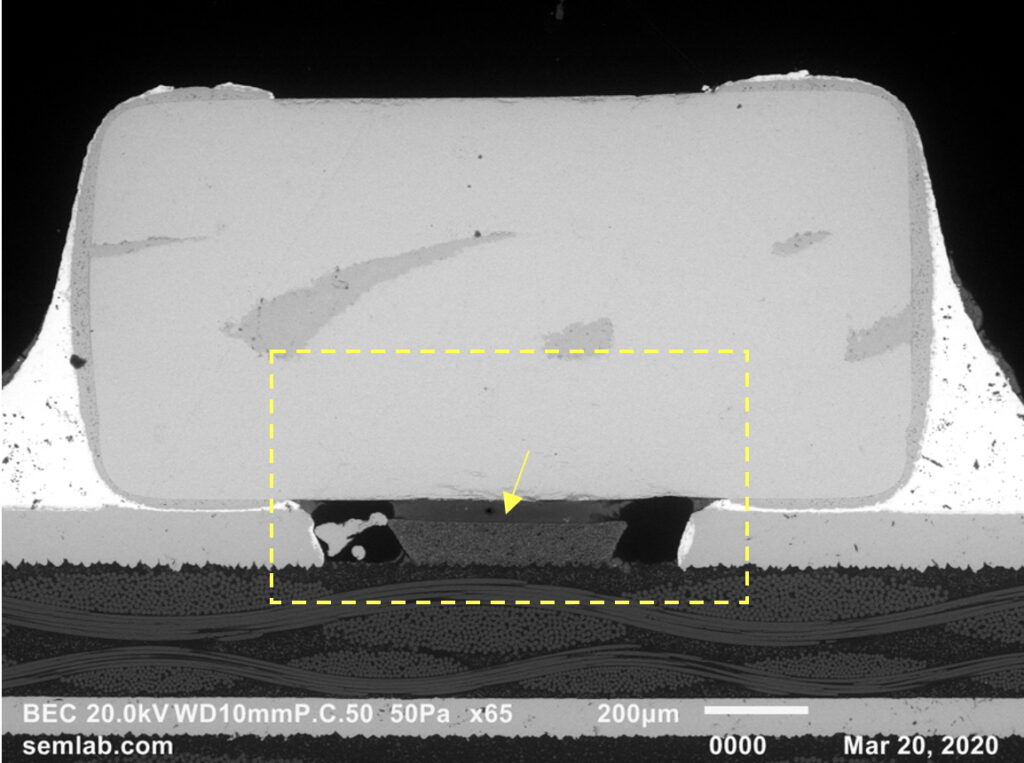
An EDS map for Sn (Fig. B) shows a large concentration of tin below the capacitor and above the solder mask. This indicates that an electrochemical cell existed under the capacitor allowing tin to migrate from the anode to the cathode. The PCBA was operating in a moist environment, which is a contributing factor.
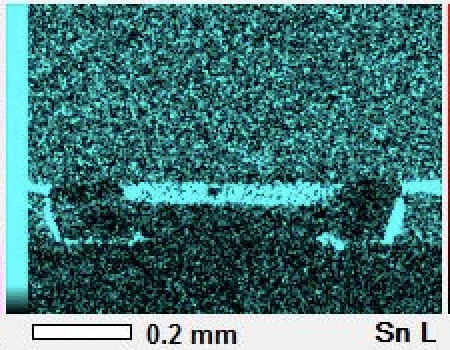
A second way we can look at the problem is to mechanically excavate the solder joints and remove the capacitor from the PCBA so that residue and corrosion damage can be observed on the board surface under the capacitor (Fig. C) and on the bottom surface of the capacitor (Fig. D).
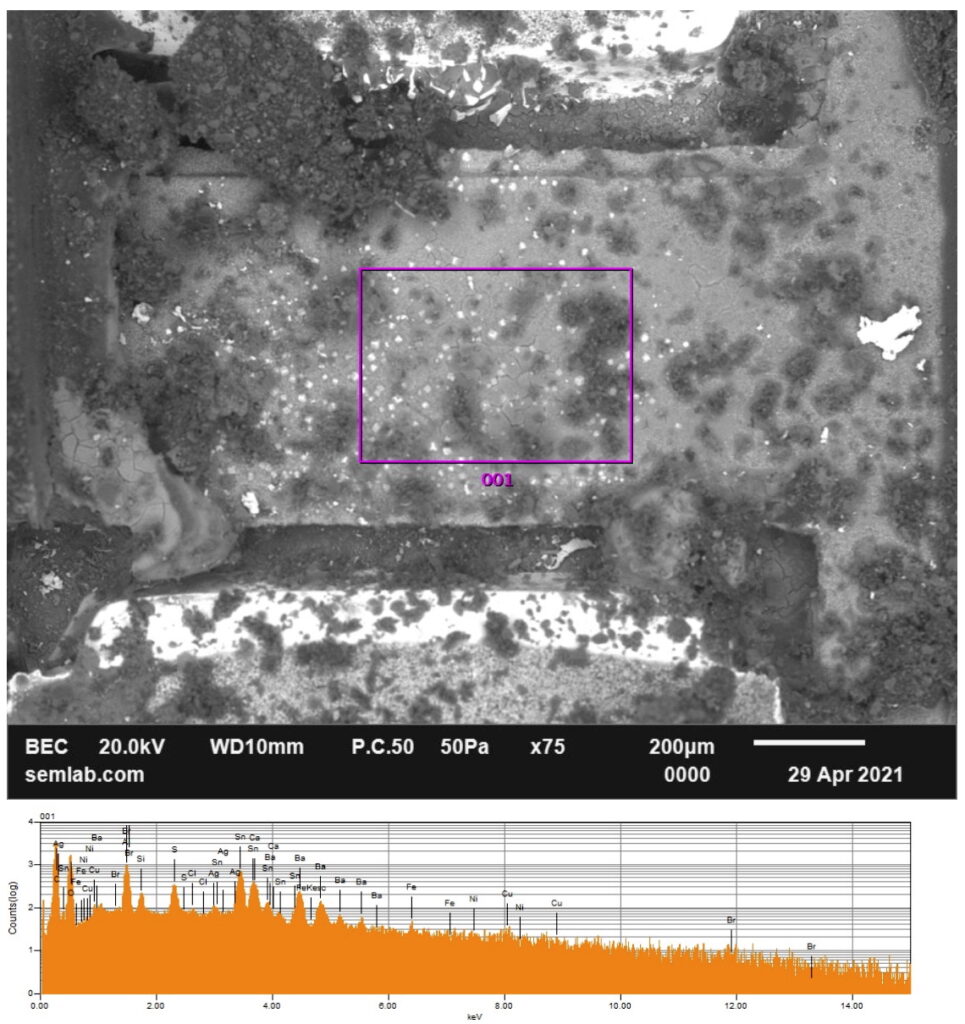
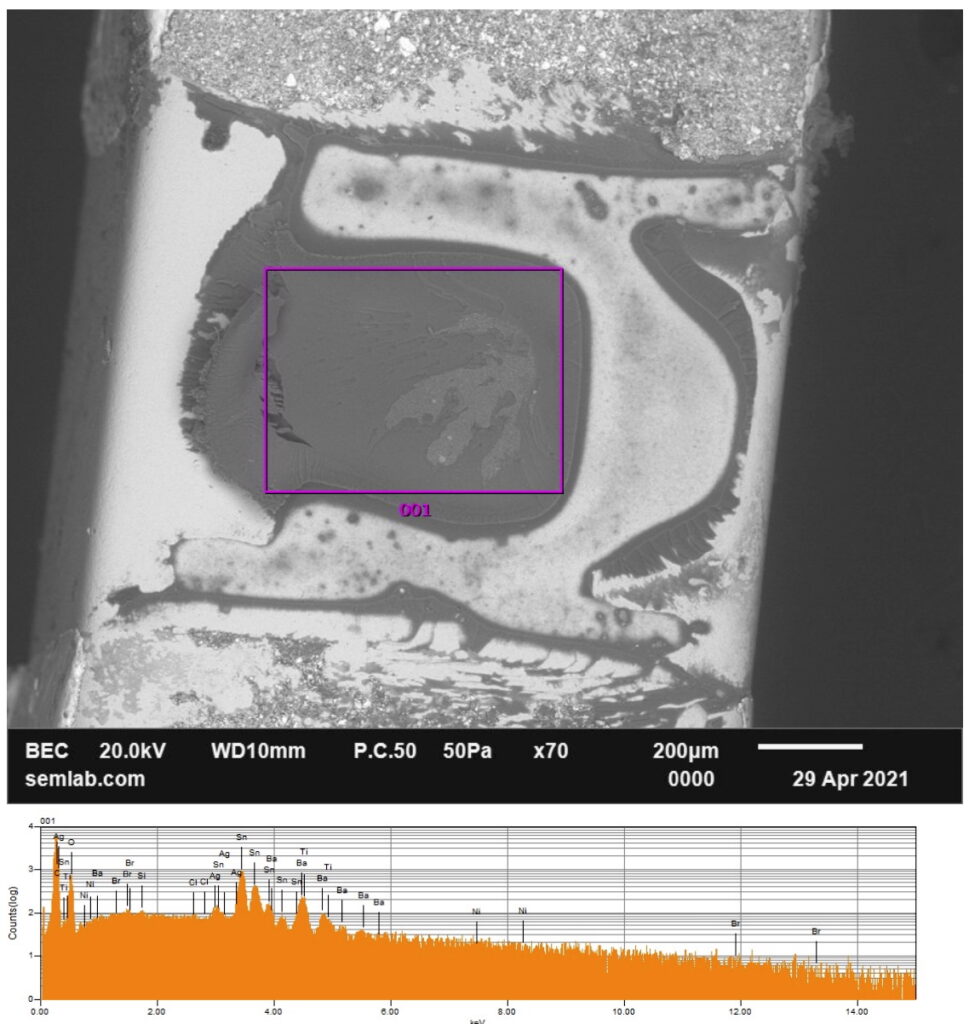
Electrochemical corrosion and electromigration can involve more than corrosion of tin, as we also see corrosion of silver, copper, and nickel plating associated with metal end caps and PWB mounting pads or from SAC305 solder.
Contamination by chlorine and bromine flux activators is common and accelerates the rate of corrosion.
This is how it works …
- Residual solder flux absorbs moisture from the environment, creating an ionic solution under the MLCC.
- Under applied voltage, metals such as silver (Ag), tin (Sn), nickel (Ni) or copper (Cu) from electrodes or solder pads ionize.
- Metal cations migrate toward the cathode.
- At the cathode, metal ions are reduced and deposited as metallic dendrites.
- Dendrites grow until they bridge the gap between electrodes, creating a short circuit.
Even if the corrosion process doesn’t create a hard metallic short, the generation of ionic species under the capacitor can cause large enough leakage currents to generate circuit faults.
Time to failure is a function of many parameters including quantity and type of solder flux residues, operating temperature, moisture level, and electric field magnitude. The electric field becomes a significant factor as capacitor size decreases. As MLCC sizes decrease, the gap between electrodes (spacing under the component) also shrinks so that the electric field is higher.
So my question to circuit designers is …
How do you incorporate these considerations into your design rules?